Proteins are essential biomolecules that perform a vast array of functions within living organisms. They act as enzymes, antibodies, messengers, structural components, transporters, receptors, and more. The development of recombinant DNA technology in the 1970s enabled scientists to produce specific proteins for research, industrial and pharmaceutical applications.
This involves isolating the DNA sequence encoding the protein, inserting it into an expression vector, transfecting the vector into host cells, growing cell cultures to express the protein, and finally purifying the protein. The process from gene identification to purified protein is a complex, multi-step pipeline.
This article will provide an overview of the key stages of recombinant protein production. Understanding the protein production pipeline is important for researchers looking to generate proteins for structure-function studies, industry seeking to manufacture therapeutic proteins or enzymes, and anyone interested in learning more about how recombinant technology allows us to harness bacterial and mammalian cells to produce functional human proteins.
Page Contents
Gene Identification and Isolation
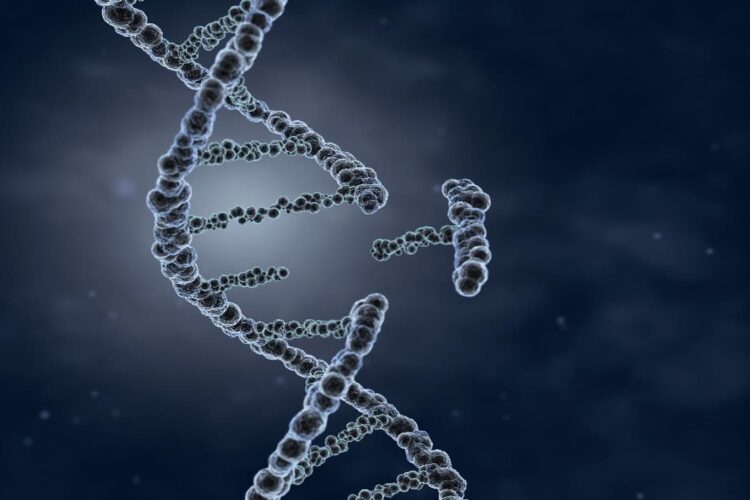
Source: pioneeringminds.com
The first step is to identify the gene encoding the protein of interest. The human genome contains around 20,000 protein-coding genes. Bioinformatic approaches can be utilized to search genomic and transcriptomic databases to find the gene sequence. Alternatively, if the protein’s amino acid sequence is known, the gene can be reverse-translated to obtain the coding DNA sequence.
Once the gene is identified, it can be isolated from a DNA sample through polymerase chain reaction (PCR) amplification using synthetic oligonucleotide primers complementary to the gene’s flanking sequences. The PCR product is then inserted into a cloning vector like a plasmid using DNA ligase enzyme to create a recombinant DNA molecule. Plasmids are circular DNA molecules that replicate independently within host cells. The gene insert can be sequenced to confirm its identity and fidelity before proceeding further.
Vector Design and Construction
The choice of the vector influences the yield and properties of the recombinant protein ultimately obtained. Important vector elements include the origin of replication, antibiotic resistance gene, promoter, multiple cloning site, and terminator. The origin of replication determines the vector copy number per cell. High copy vectors lead to greater yields but can overtax the cellular machinery. Antibiotic resistance provides selection for cells containing the vector.
The promoter initiates transcription of the target gene, so a strong constitutive or inducible promoter optimizes expression. The multiple cloning site provides restriction sites for gene insertion. Common plasmids used include pUC, pBR322, and pET systems. Viral vectors based on adenovirus, lentivirus or baculovirus can also be engineered. Optimal vector design ensures efficient transcription and translation of the gene insert in the host cell.
Host Cell Transformation
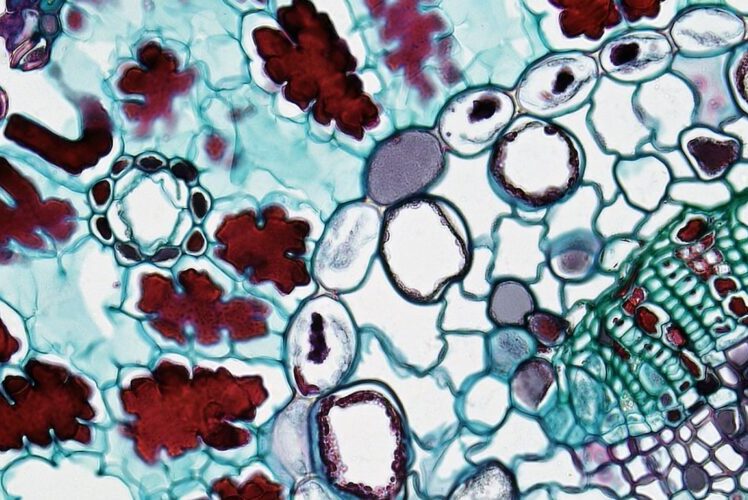
Source: biomatik.com
The recombinant vector is then used to genetically transform host cells. Adding the vector to E. coli or yeast cells leads to natural uptake of the plasmid. For mammalian cells like CHO or HEK293, transfection reagents or electroporation create temporary pores in the cell membrane enabling vector entry. Viral transduction is also highly efficient for gene delivery and integration in many cell types.
Transformed cells are grown on selective media and screened for the presence of the vector. Integrating vectors can achieve stable long-term target gene expression. Non-integrating vectors lead to temporary expression until the plasmid is lost through cell division. Host cells are chosen based on their ability to accurately transcribe, translate and process the protein of interest. Matching the vector and host optimizes production.
Protein Expression
Culturing transformed host cells leads to transcription of the target gene into mRNA and subsequent translation into the encoded protein. Expression is optimized by adjusting parameters like cell density, culture medium, temperature, transfection efficiency and timing. Strong promoters and high copy vectors lead to overexpression of the protein. Using an inducible promoter allows tight regulation of protein expression, often leading to higher purity and reduced toxicity.
Autoinduction systems utilizing lactose for plasmid uptake and inducer exclusion improve protein yields. Shaking and aeration parameters in bacterial cultures enhance oxygenation for better growth and protein synthesis. Manipulating culture duration and inducer concentrations modifies expression levels. Various tags like 6xHis, FLAG or Strep can also be incorporated to aid downstream purification. The goal is to achieve the highest yields of soluble, correctly folded protein.
For those intrigued about delving deeper into the technicalities and nuances of such services, there’s a webpage about expression services that encapsulate this concisely.
Protein Isolation and Purification
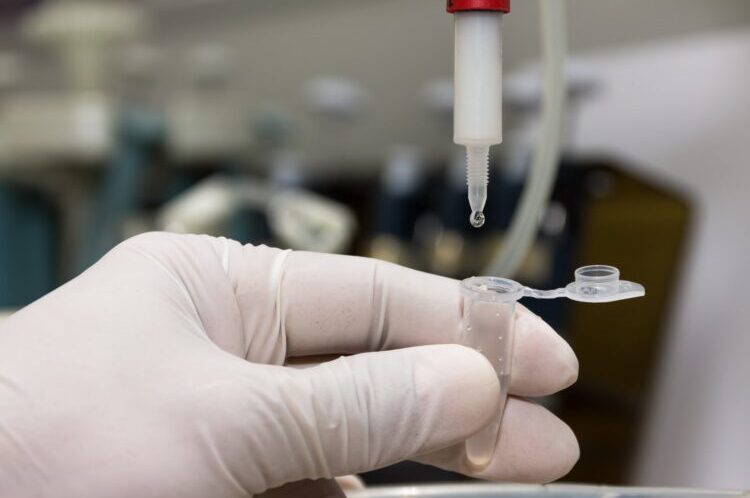
Source: sepmag.eu
After expression, the target protein must be recovered and purified from the raw lysate which contains cellular proteins, nucleic acids, lipids and metabolites alongside the recombinant protein. First cells are harvested from culture by centrifugation and lysed by mechanical shearing or enzymatic digestion. Initial purification utilizes the differences in protein solubility, size, charge and binding affinity to remove contaminants.
Common techniques include ammonium sulfate precipitation, centrifugation, microfiltration, packed bed chromatography, electrophoresis, liquid-liquid extraction and affinity purification. Combining multiple strategies results in higher purity. His-tagged proteins are purified using nickel resin columns. Antibody affinity purification can isolate specific antigens. Additional polishing steps like dialysis, endotoxin removal and concentration are used to obtain the final purified protein.
Analysis and Validation
Various analytical methods assess the purity, integrity, activity and overall quality of the purified protein. SDS-PAGE electrophoresis combined with Coomassie or silver staining provides size estimation and purity profiling. Western blots with tag or target-specific antibodies confirm protein identity. Peptide mass fingerprinting utilizes proteolytic digestion and mass spectrometry to identify proteins in a sample.
Circular dichroism spectroscopy characterizes protein secondary structure and folding. Enzymatic assays directly measure protein function and activity levels. Liquid chromatography techniques quantitate aggregate or degraded species. Endotoxin assays validate removal. Once fully validated, the purified proteins can proceed to downstream applications. Thorough analytical testing ensures the protein product matches design specifications.
Applications of Recombinant Proteins
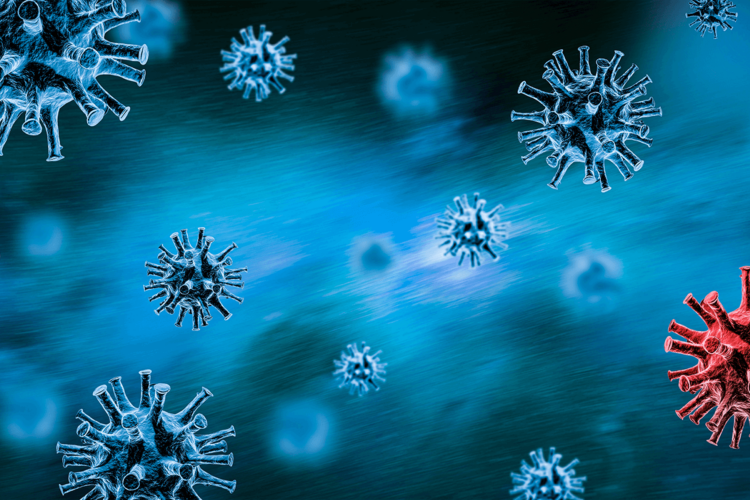
Source: blog.cellsignal.com
Recombinant proteins have become indispensable tools in both basic research and biotechnology industries. Producing proteins in heterologous systems allows generation of better antibodies as immunogens. tagged proteins aid co-immunoprecipitation studies to uncover protein-protein interactions and networks. Enzymes are manufactured for industrial and pharmaceutical uses.
Biopharmaceuticals like insulin, growth factors and antibodies employ recombinant technology for cost-effective production. Recombinant proteins also facilitate structure-function analyses through site-directed mutagenesis. Studying recombinant proteins provides deeper insight into biological processes. The customizability of recombinant protein production enables a vast array of research and commercial applications.
The ability to produce functional, purified proteins from cloned genes has transformed scientific capabilities and biomedical applications. While the underlying process from gene isolation to protein purification involves multiple complex steps, recombinant technology makes it readily feasible to generate a diverse array of proteins. Continued advances in gene synthesis, genome editing, transfection efficiencies, protein engineering, bioprocessing and purification technologies will further expand the utility of recombinant proteins to meet emerging needs in medicine, biotechnology and basic research.